Ichthyotoxic Cochlodinium polykrikoides red tides offshore in the South Sea, Korea in 2014: III. Metazooplankton and their grazing impacts on red-tide organisms and heterotrophic protists
Article information
Abstract
Cochlodinium polykrikoides red tides have caused great economic losses in the aquaculture industry in many countries. To investigate the roles of metazooplankton in red tide dynamics of C. polykrikoides in the South Sea of Korea, the abundance of metazooplankton was measured at 60 stations over 1- or 2-week intervals from May to November 2014. In addition, the grazing impacts of dominant metazooplankton on red tide species and their potential heterotrophic protistan grazers were estimated by combining field data on the abundance of red tide species, heterotrophic protist grazers, and dominant metazooplankton with data obtained from the literature concerning ingestion rates of the grazers on red tide species and heterotrophic protists. The mean abundance of total metazooplankton at each sampling time during the study was 297–1,119 individuals m−3. The abundance of total metazooplankton was significantly positively correlated with that of phototrophic dinoflagellates (p < 0.01), but it was not significantly correlated with water temperature, salinity, and the abundance of diatoms, euglenophytes, cryptophytes, heterotrophic dinoflagellates, tintinnid ciliates, and naked ciliates (p > 0.1). Thus, dinoflagellate red tides may support high abundance of total metazooplankton. Copepods dominated metazooplankton assemblages at all sampling times except from Jul 11 to Aug 6 when cladocerans and hydrozoans dominated. The calculated maximum grazing coefficients attributable to calanoid copepods on C. polykrikoides and Prorocentrum spp. were 0.018 and 0.029 d−1, respectively. Therefore, calanoid copepods may not control populations of C. polykrikoides or Prorocentrum spp. Furthermore, the maximum grazing coefficients attributable to calanoid copepods on the heterotrophic dinoflagellates Polykrikos spp. and Gyrodinium spp., which were grazers on C. polykrikoides and Prorocentrum spp., respectively, were 0.008 and 0.047 d−1, respectively. Therefore, calanoid copepods may not reduce grazing impact by these heterotrophic dinoflagellate grazers on populations of the red tide dinoflagellates.
INTRODUCTION
Metazooplankton including copepods, cladocerans, chaetognaths, larvae of invertebrates, and hydrozoans are a major component of the marine ecosystem (Fulton 1984, Kang et al. 1996, Uye and Liang 1998, Calbet 2001, Gallienne and Robins 2001, Puelles et al. 2003, Kimmel and Roman 2004, Turner 2004, Tseng et al. 2009). They are consumers of phytoplankton and heterotrophic protists and are in turn prey for larval fish and other metazoans (Porter et al. 1985, Houde and Roman 1987, Turner et al. 1988, Stoecker and Capuzzo 1990, Sanders and Wickham 1993, Carlsson et al. 1995, Croll et al. 2005, Jeong et al. 2007, Waggett et al. 2008, Lazareva and Kopylov 2011). Thus, the dynamics of metazooplankton affect the population dynamics of diverse marine organisms.
Red tides or harmful algal blooms are caused by exclusively autotrophic, mixotrophic, and heterotrophic protists (Smayda 1997, Jeong et al. 2010, 2013, 2015, Lee et al. 2015, Yoo et al. 2015, Aktan and Keskin 2017) and often cause great economic losses in the aquaculture and tourism industries (Smayda 1990, Glibert et al. 2005, Anderson et al. 2012, Fu et al. 2012). Thus, understanding the processes of red tides and predicting their outbreak, persistence, and decline are needed to minimize loss (e.g., Jeong et al. 2015). There have been many studies on the feeding of metazooplankton on red tide organisms, but fewer studies on the grazing impacts of metazooplankton on red tide organisms (Uye 1986, Turner and Granéli 1992, Turner and Tester 1997, Calbet et al. 2003). Thus, it is worthwhile to explore the effects of metazooplankton on red tide organisms in natural environments. In general, many heterotrophic protists are effective grazers on red tide organisms and are in turn prey for metazooplankton (Houde and Roman 1987, Jeong and Latz 1994, Carlsson et al. 1995, Jeong 1999, Jeong et al. 1999, 2001, 2003, Kim and Jeong 2004, Tillmann 2004, Cohen et al. 2007, Yoo et al. 2010, 2013b, Park et al. 2013). An assessment of predation impacts of metazooplankton on populations of heterotrophic protists that graze on red tide organisms is needed to understand the dynamics and interactions among these three components.
Red tides frequently occur in the South Sea of Korea where there is a high concentration of aquacages (Jeong et al. 2013, 2017, Kim et al. 2013a, Lim et al. 2017). These red tides often cause a great loss in the aquaculture industry (e.g., Park et al. 2013). The causative species for red tides in this region are Cochlodinium polykrikoides, Prorocentrum spp., Alexandrium spp., Ceratium spp., Karenia spp., and diatoms (e.g., Jeong et al. 2017). In particular, red tides dominated by C. polykrikoides have caused 1–60 M US dollars-worth of damage (Park et al. 2013). Thus, predicting the process of red tides is a critical step in minimizing damage. There have been several studies on the effects of physical, and chemical, biological environmental factors on the growth of C. polykrikoides (Jeong et al. 2004, 2008, 2017, Kim et al. 2004, Oh et al. 2006, Lim et al. 2014, 2017). However, few studies have investigated the grazing impact by metazooplankton on C. polykrikoides (Kim 2005, Kim et al. 2013a). Therefore, it is worthwhile to explore this topic.
In the present study, the abundance of metazooplankton was measured at 60 stations over 1- or 2-week intervals, from May to November 2014, to investigate the roles of metazooplankton in the red tide dynamics of C. polykrikoides in the South Sea of Korea. In addition, the grazing impacts of dominant metazooplankton on red tide species and their potential heterotrophic protistan grazers were estimated by combining field data on the abundance of red tide species, heterotrophic protist grazers, and dominant metazooplankton with data obtained from the literature concerning ingestion rates of the grazers on red tide species and heterotrophic protists. The results of the present study provide a basis for understanding the interactions among red tide organisms, heterotrophic protists, and metazooplankton, and help understand the roles of metazooplankton in red tide dynamics.
MATERIALS AND METHODS
Study area
Plankton samples were collected from 60 sampling stations located centrally along the southern coast of the Korean peninsula between Goheung to Tongyoung (approximately 140 km × 40 km, 34.3° E-34.8° E and 127.3° N-128.4° N) (Fig. 1). The water depth for all sampling stations ranged from 4–53 m. The stations in the western area were shallower than those in the eastern area at the same latitude.
Sampling and analyses of physicochemical and biological properties
Sixteen sampling cruises were carried out at 1–2-week intervals between May 7 and Nov 10, 2014 (Fig. 2). A 2-day sampling was carried out by two ships as in Jeong et al. (2017) and Lim et al. (2017). On the first day, water samples were collected from one ship at stations 101–115, whereas the other ship collected samples at stations 201–309. On the second day, one ship collected water samples at stations 501–507 and 601–608, whereas the other ship collected at stations 701–703, 801–805, and 901–904. The sampling time at the first station in each sampling day was between 07:30–08:00 h and at the last station between 14:00–15:00 h.
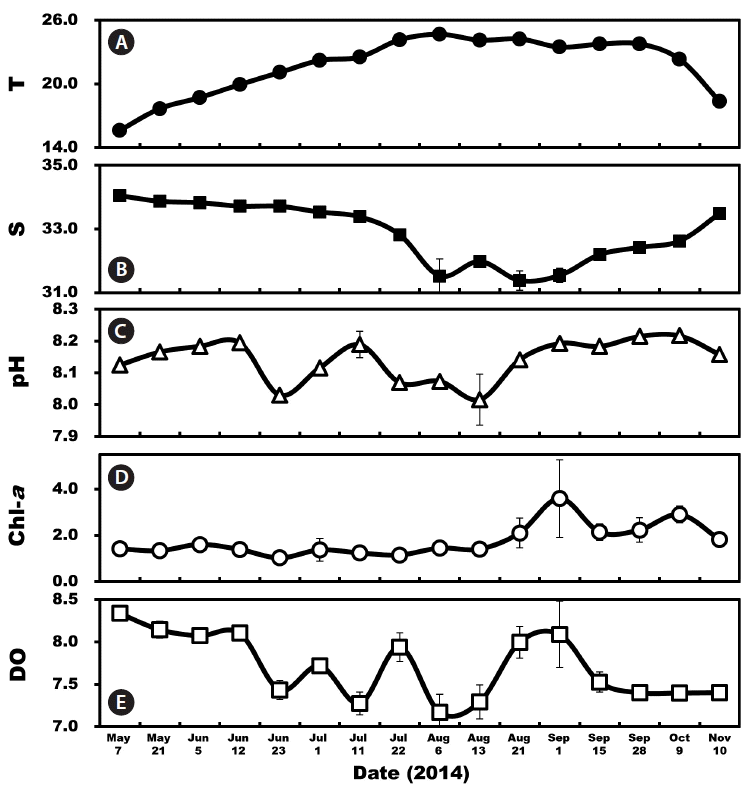
Environmental factors in the South Sea of Korea from May 7 to Nov 10, 2014. (A) Average water temperature (T, °C). (B) Average salinity (S). (C) pH. (D) Average chlorophyll-a concentrations (Chl-a, mg m−3). (E) Dissolved oxygen (DO, mg L−1). Symbols represent average ± standard error.
Water temperature and salinity in the water column were measured using two CTDs (YSI6600; YSI Inc., Yellow Springs, OH, USA and Ocean seven; Idronaut S.r.l., Milan, Italy). The data obtained from the CTDs were calibrated in each cruise. Additionally, the temperature, salinity, pH, and dissolved oxygen (DO) for each sampling depth were measured using a YSI Professional Plus instrument (YSI Inc.). The chlorophyll-a concentration (Chl-a) was measured as described in American Public Health Association (1995).
Metazooplankton samples were collected at every station by towing a 303 μm mesh, 45 cm diameter conical plankton net with a flowmeter vertically from the bottom to the surface (or 30 m if the bottom depth was more than 30 m) every sampling interval from May to November 2014. Each plankton sample was poured into a 500-mL polyethylene bottle and preserved with 4% formalin. Species identification and determination of metazooplankton abundance was performed using dissecting and inverted microscopes at magnifications of ×40 and ×200.
Data on phytoplankton, including red tide species and heterotrophic protists, were obtained from Jeong et al. (2017) and Lim et al. (2017) in which sampling was conducted at the same times and stations as in this study.
Grazing impact
The calanoid copepods Acartia spp. are known to feed on various species of dinoflagellates such as Cochlodinium polykrikoides, Prorocentrum donghaiense, Polykrikos kofoidii, and Oxyrrhis marina (Jeong et al. 2001, Kim 2005). Thus, it was assumed that the ingestion rate of Acartia spp. on dinoflagellates C. polykrikoides, P. donghaiense, P. kofoidii, and O. marina were the same as the ingestion rate of total calanoid copepods on the C. polykrikoides, Prorocentrum spp., P. kofoidii, and Gyrodinium spp., respectively.
The grazing coefficients (g, d−1) were calculated as
where CR (mL predator−1 h−1) is the clearance rate of the predator on a target prey at a prey concentration and PC is a predator concentration (predator mL−1). The CR values were calculated as
where IR (cells eaten predator−1 h−1) is the ingestion rate of a predator on the target prey and X (cells mL−1) is the prey concentration. These CR values were corrected using Q10 = 3.2 (Hansen et al. 1997) because the in-situ water temperature and the temperature used in the laboratory for this experiment (20–22°C) were sometimes different.
Data process
The spatiotemporal distributions of each taxon of metazooplankton communities were plotted by Surfer (Golden software, LLC, Golden, CO, USA). The correlation coefficients between physical, chemical, and biological properties were calculated using the Pearson’s correlation (Conover 1980, Zar 1999). By combining field data on the abundance of predator and prey species with the ingestion rates of the predator on the prey obtained in the literatures with some assumptions, we estimated the grazing coefficients.
RESULTS
Environmental properties
The mean surface water temperature during the study varied from 15.6 to 24.7°C with the highest temperature occurring in August 2014 and the lowest temperature in May (Fig. 2A). The mean surface salinity ranged from 31.4 to 34.1 with the highest salinity occurring in May and the lowest salinity in August (Fig. 2B). The pH ranged from 8.02 to 8.22 and the Chl-a from 1.0 to 3.6 μg L−1 with a peak on Sep 1 (Fig. 2C & D). DO ranged from 7.2 to 8.3 mg L−1 (Fig. 2E).
Species composition of metazooplankton
During the study 23 copepods, 4 cladocerans, 10 invertebrate larva, and 10 other metazooplankton taxa were found (Appendix 1). These included the copepods Acartia erythraea, A. omorii/hongi, Calanopia thompsoni, Calanus sinicus, Centropages abdominalis, Centropages tenuiremis, Corycaeus affinis, Euchaeta sp., Labidocera euchaeta, Labidocera rounta, Labidocera sp., Neocalanus sp., Oithona similis, Oithona sp., Oncaea sp., Paracalanus parvus, Pseudodiaptomus marinus, Sinocalanus tenellus, Temora turbinata, Temora discaudata, Tortanus forcipatus, harpacticoid copepods, and copepodites, the cladocerans Evadne nordmanni, E. tergestina, Penilia avirostris, and Podon polyphemoides. In addition, barnacle larvae, decapod zoea, chaetognaths, siphonophores, and appendicularians were observed.
Of these metazooplankton, A. omorii/hongi, C. affinis, barnacle nauplii, decapod zoea, decapod mysid, echinoderm larvae, gastropod larvae, polychaete larvae, chaetognaths, hydromedusa, siphonophores, amphipods, and appendicularians were present at all sampling times (Appendix 1). However, C. abdominalis, Neocalanus sp., S. tenellus, and E. nordmanni were only present during one sampling.
Abundance of metazooplankton
The abundance of total metazooplankton at each sampling during this study were 1–13,131 individuals (inds.) m−3 and mean abundance was 297–1,119 inds. m−3 (Table 1, Fig. 3). The mean abundance of total metazooplankton was high from Jun 23 to Aug 13 (Fig. 3B).

The range of abundance of total metazooplankton and each taxon, and water temperatures and salinity where each taxon was found in the South Sea of Korea from May to November 2014
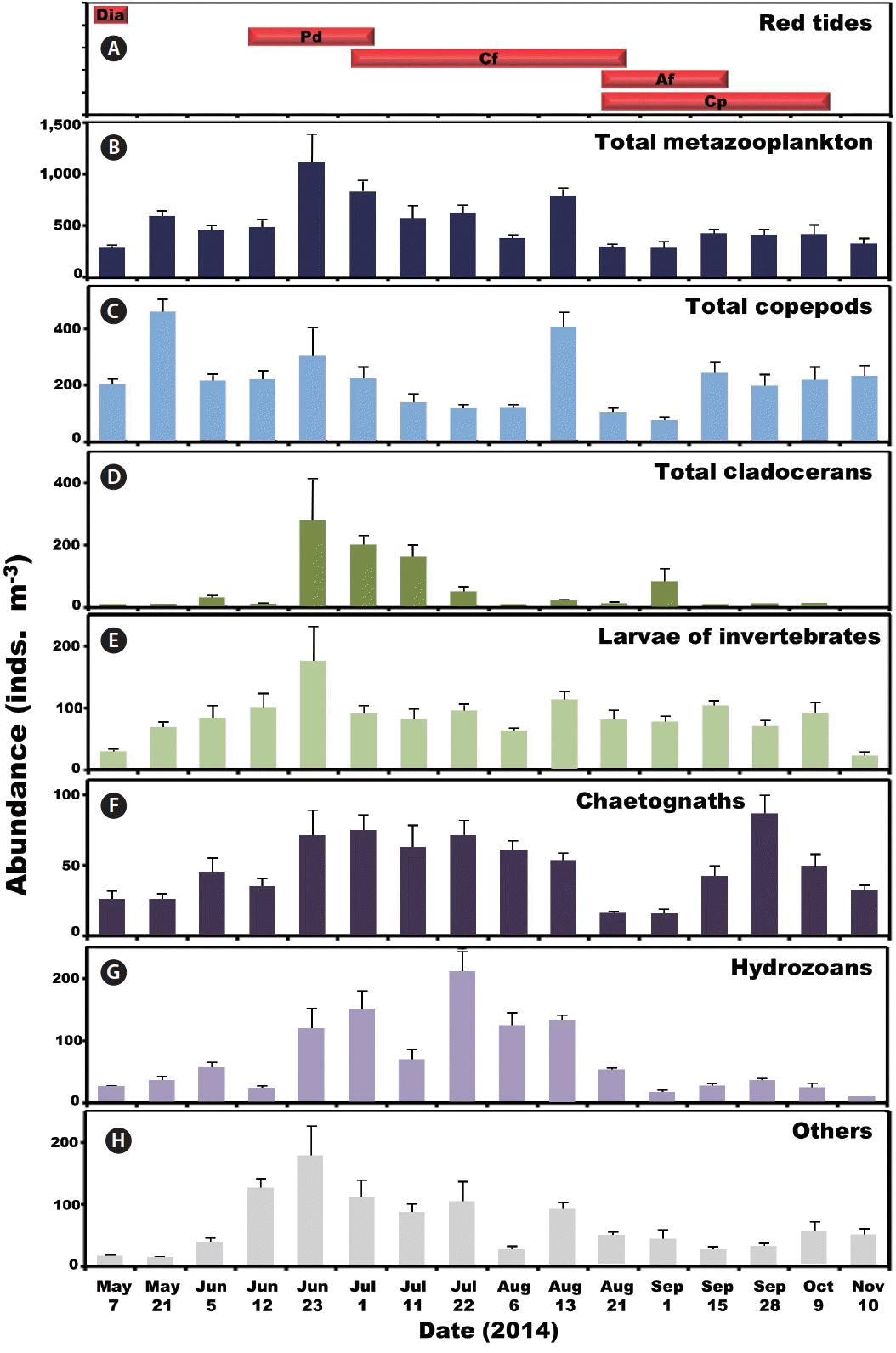
The period of red tides and abundance (inds. m−3) of metazooplankton taxa. (A) The period of red tides dominated by diatoms (Dia), Prorocentrum donghaiense (Pd), Ceratium furca (Cf), Alexandrium fraterculus (Af), and Cochlodinium polykrikoides (Cp). Average abundance of total metazooplankton (B), total copepods (C), total cladocerans (D), larvae of invertebrates (E), chaetognaths (F), hydrozoans (G), and others (H). Bars represent average ± standard error.
Copepods dominated metazooplankton assemblages at all sampling times except from Jul 11 to Aug 6 when cladocerans and hydrozoans dominated (Fig. 3). The maximum mean abundance of copepods was 453 inds. m−3 on May 21. Furthermore, cladocerans and benthic invertebrate larvae dominated metazooplankton assemblages on Jun 23 with the maximum mean abundance of cladocerans and benthic invertebrate larvae being 274 and 178 inds. m−3, respectively (Fig. 3). Moreover, chaetognaths, hydrozoans, and other metazooplankton taxa were abundant from Jun 23 to Aug 13 (Fig. 3). The maximum mean abundance of chaetognaths, hydrozoans, and other metazooplankton was 87, 210, and 178 inds. m−3, observed on Sep 28, Jul 22, and Jun 23, respectively (Fig. 3).
Spatiotemporal distributions of the total metazooplankton
Metazooplankton predominantly inhabited the marginal and shallower regions (<30 m depth) of the study area. From May 7 to Aug 13, metazooplankton occurred in high concentrations (<13,200 inds. m−3) near the shallow waters of Goheung and Yeosu (Fig. 4). In contrast, on Sep 1, metazooplankton were regionally concentrated (<2,700 inds. m−3) in the shallow waters of Tongyoung, and abundance of total metazooplankton was high (<1,800 inds. m−3) in the shallow waters of Goheung from Sep 15 to 27 (Fig. 4). Furthermore, metazooplankton were distinctively more concentrated (<1,900 inds. m−3) in the offshore region (>30 m depth) than the shallow waters in November (Fig. 4). In general, the distributions of total copepods and larvae of invertebrates were similar to that of total metazooplankton (Figs 4 & 5).

Distributions (inds. m−3) of total metazooplankton (TMZ), total copepods (TCop), and total cladocerans (TCla) in the study area for each cruise from May 7 to Nov 10, 2014.
Correlations between abundance of major metazooplankton taxa and environmental factors
The abundance of total metazooplankton was significantly positively correlated with the concentration of total phototrophic dinoflagellates, but negatively correlated with pH (Table 2). The abundance of copepods was significantly positively correlated with salinity, but negatively correlated with water temperature (T), pH, and DO (Table 2). Moreover, the abundance of cladocerans was significantly positively correlated with DO (Table 2).

Correlations between the abundance of metazooplankton taxon and physical, chemical, and biological factors in the South Sea of Korea from May to November 2014
The abundance of invertebrate larvae was significantly positively correlated with T, Chl-a, and abundance of phototrophic dinoflagellates and tintinnid ciliates (TCI) but negatively correlated with salinity and pH (Table 2). Furthermore, the abundance of the chaetognaths was significantly positively correlated with T, but negatively correlated with salinity, pH, and DO (Table 2). However, the abundance of chaetognaths significantly positively correlated with Chl-a and TCI (Table 2). In addition, the abundance of the hydrozoans was significantly positively correlated with temperature, but negatively correlated with pH (Table 2).
At the species level, the abundance of C. sinicus, echinoderm larvae, and siphonophores was significantly positively correlated with the abundance of total diatoms, whereas the abundance of C. affinis, barnacle nauplii, decapod zoea, echinoderm larvae, fish larvae, and siphonophores was significantly positively correlated with the abundance of the diatom Pseudo-nitzschia spp. (Table 3). Furthermore, the abundance of C. affinis, L. euchaeta, L. rotunda, barnacle nauplii, decapod zoea, fish larvae, hydromedusa and siphonophores was significantly positively correlated with that of total phototrophic dinoflagellate (Table 4). In addition, the abundance of C. affinis, L. euchaeta, L. rotunda, P. parvus, P. avirostris, barnacle nauplii, decapod zoea, fish larvae, chaetognaths, hydromedusa, and siphonophores was significantly positively correlated with that of the dinoflagellate P. donghaiense (Table 4). The abundance of C. affinis, L. euchaeta, L. rotunda, P. parvus, copepodite, P. avirostris, barnacle nauplii, decapod zoea, echinoderm larvae, fish larvae, chaetognaths, hydromedusa, and siphonophores was significantly positively correlated with that of the tintinnid ciliate Tintinnopsis tubulosoides (Table 5). Finally, the abundance of salpids was significantly negatively correlated with that of T. tubulosoides (Table 5).

Correlations between the abundance of metazooplankton taxa and the abundance of potential diatom prey species in the South Sea of Korea from May to November 2014
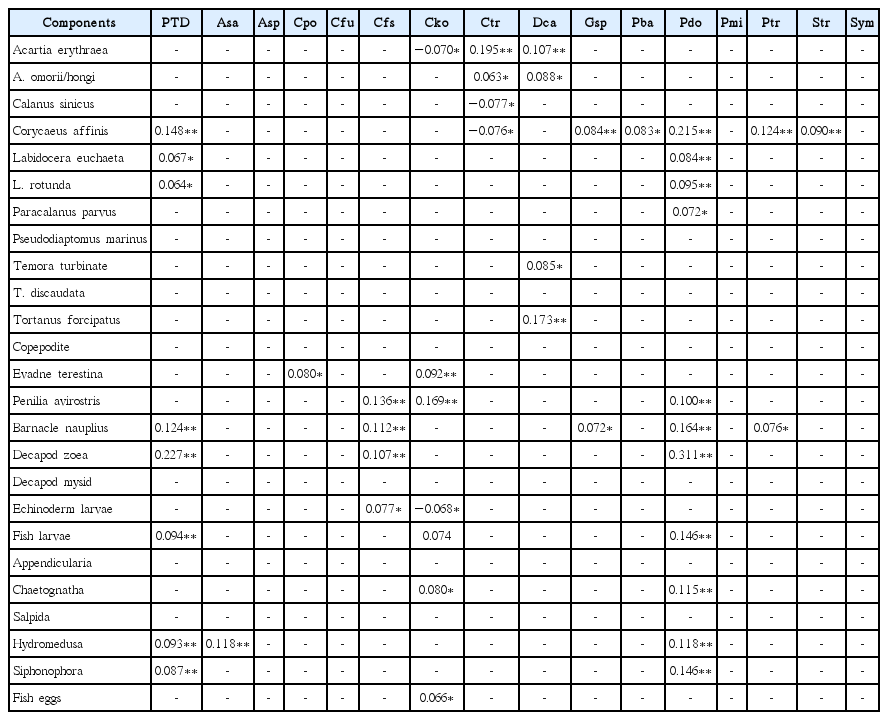
Correlations between the abundance of metazooplankton taxa and the abundance of potential phototrophic dinoflagellate prey species in the South Sea of Korea from May to November 2014
Grazing impact by calanoid copepods on red-tide organisms and heterotrophic dinoflagellates
When the abundance of the phototrophic dinoflagellate C. polykrikoides and co-occurring calanoid copepods was 1–2,990 cells mL−1 and 1–2,480 inds. m−3, respectively, the calculated grazing coefficient attributable to calanoid copepods on co-occurring C. polykrikoides was up to 0.018 d−1 (Jeong et al. 2017) (Fig. 6). Furthermore, when the abundance of the phototrophic dinoflagellate Prorocentrum spp. and co-occurring calanoid copepod species was 0.2–2,725 cells mL−1 and 0.9–1,076 inds. m−3, respectively, the calculated grazing coefficients attributable to total calanoids on co-occurring Prorocentrum spp. were 0.001–0.029 d−1 (Jeong et al. 2017) (Fig. 7).
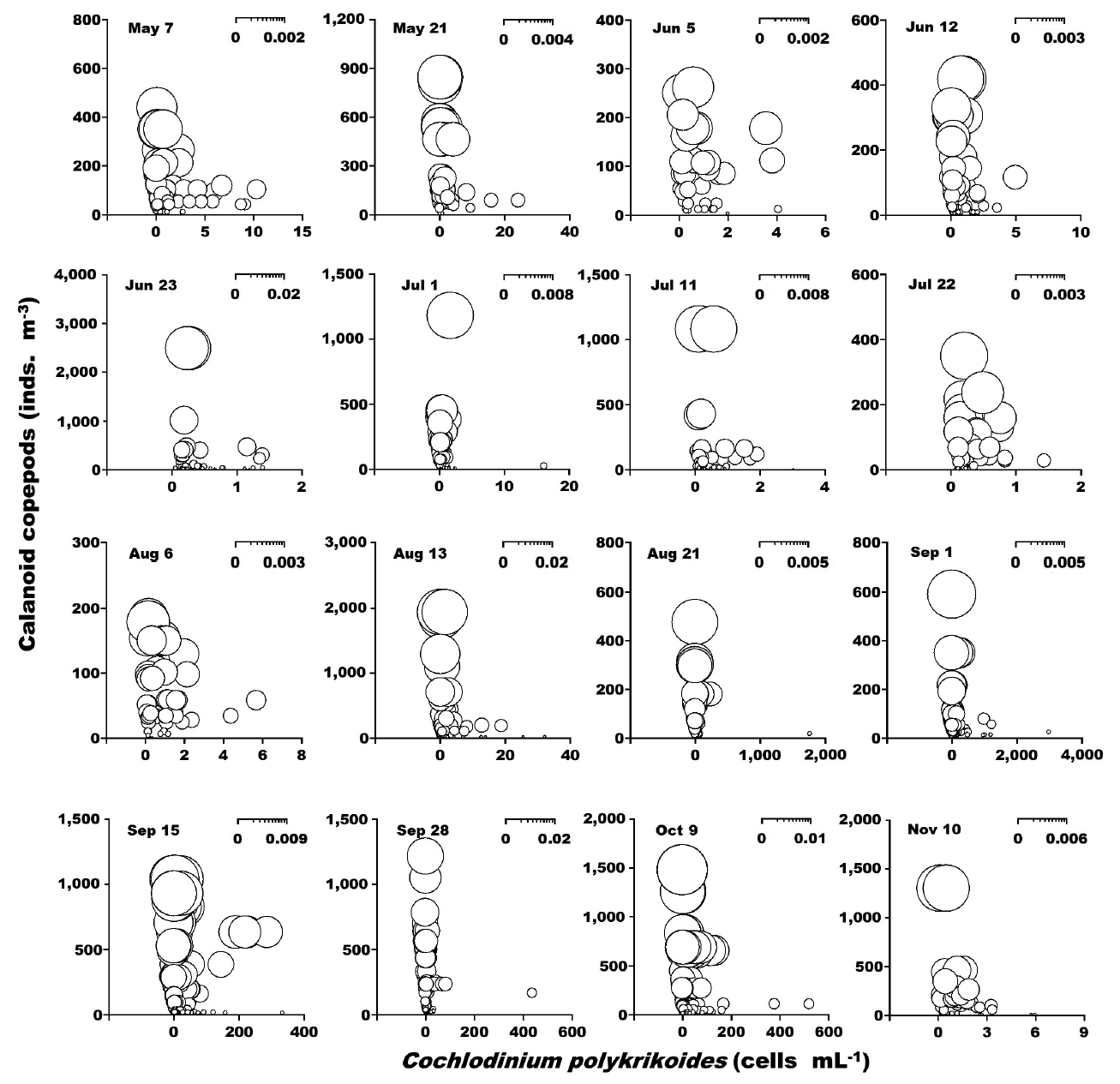
Calculated grazing coefficients (g, d−1) attributable to calanoid copepods on co-occurring Cochlodinium polykrikoides, based on assumption (see text) in the study area for each cruise from May 7 to Nov 10, 2014.

Calculated grazing coefficients (g, d−1) attributable to calanoid copepods on co-occurring Prorocentrum spp., based on assumption (see text) in the study area at each cruise from May 7 to Nov 10, 2014.
When the abundance of the heterotrophic dinoflagellates Gyrodinium spp. and co-occurring calanoid copepods was up to 42 cells mL−1 and 2,940 inds. m−3, respectively, the calculated grazing coefficients attributable to calanoid copepods on co-occurring Gyrodinium spp. were 0.0003–0.047 d−1 (Lim et al. 2017) (Fig. 8). When the abundance of the heterotrophic dinoflagellates Polykrikos spp. and co-occurring calanoid copepods was 0.02–79 cells mL−1 and up to 1,035 inds. m−3, respectively, the calculated grazing coefficients attributable to calanoid copepods on co-occurring Polykrikos spp. were 0.0001–0.008 d−1 (Lim et al. 2017) (Fig. 9).

Calculated grazing coefficients (g, d−1) attributable to calanoid copepods on co-occurring heterotrophic dinoflagellates Gyrodinium spp., based on assumption (see text) in the study area for each cruise from May 7 to Nov 10, 2014.
DISCUSSION
Abundance of metazooplankton
The maximum abundance of total metazooplankton obtained during this study (1.3 × 104 inds. m−3) is comparable to that in Jinhae Bay, Kangjin Bay, Seomjin River Estuary, and coastal waters of Yeosu which are located in the South Sea of Korea and Balearic Sea of Mallorca, but slightly lower than that in the Masan Bay (Korea), Newport River Estuary (UK), and NW Mediterranean (Table 6). Chl-a concentrations in the water from which the maximum abundance of total metazooplankton was obtained (2.4 μg L−1) is also comparable to that in the Seomjin River Estuary, Yeosu, NW Mediterranean, and the Balearic Sea of Mallorca, but much lower than that in Masan Bay (Calbet et al. 2001, Puelles et al. 2003, Youn et al. 2010, Kim et al. 2013a, Oh et al. 2013). Thus, in general, the maximum abundance of total metazooplankton is likely to be affected by Chl-a. Furthermore, the maximum abundance of total copepods during this study (0.4 × 104 inds. m−3) is comparable to or slightly lower than that in Kangjin Bay, coastal waters of Yeosu, the NW Mediterranean, and the Balearic Sea of Mallorca, but considerably lower than that in Masan Bay, Fukuyama Harbor (Japan), the NE Atlantic Ocean, and the Pearl River Estuary (China) (Table 6). Chl-a concentrations coinciding with the maximum abundance of copepods were much lower than that in Masan Bay and Fukuyama Harbor (Uye and Liang 1998, Kim et al. 2013a). Thus, the lower Chl-a in this study may be partially responsible for the lower maximum abundance of copepods.
Effect of environmental factors on the abundance of metazooplankton taxa
During this study, the abundance of total metazooplankton was not significantly affected by T, S, DO, and the concentrations of NO3, PO4, and SiO2. Furthermore, it was also not significantly affected by the abundance of diatoms, euglenophytes, cryptophytes, heterotrophic dinoflagellates, tintinnid ciliates, and naked ciliates. However, it was significantly affected by the abundance of phototrophic dinoflagellates. During this study, four phototrophic dinoflagellate species such as P. donghaiense, C. furca, A. fraterculus, and C. polykrikoides formed red tides (Jeong et al. 2017). Thus, dinoflagellate red tides may positively affect the abundance of total metazooplankton (Griffin et al. 2001, Turner and Borkman 2005, Jansen et al. 2006, Kim et al. 2013a).
During this study, P. donghaiense formed red tides from June to July (Jeong et al. 2017). The abundance of the copepods C. affinis, L. euchaeta, L. rotunda, P. parvus, barnacle nauplii, decapod zoea, fish larvae, chaetognaths, hydromedusa, siphonophores, and the cladoceran P. avirostris is significantly positively correlated with that of P. donghaiense. However, there have been no studies on feeding by these metazooplankton taxa on P. donghaiense and thus, it is worthwhile to explore this topic. During this study, Ceratium spp. formed red tides from July to August (Jeong et al. 2017). The abundance of the cladocerans E. tergestina and P. avirostris, barnacle nauplii, and echinoderm larvae is significantly positively correlated with that of two Ceratium species. The cladocerans E. nordmanni, P. avirostris, and Podon intermedius are known to feed on diverse algal species including Ceratium spp. (Katechakis and Stibor 2004). However, there have been no studies measuring ingestion rates of these metazooplankton taxa on Ceratium spp. and thus it is worthwhile to investigate.
Grazing impact by dominant metazooplankton on red tide organisms
In this study, calanoid copepods dominated metazooplankton assemblages at most sampling times. The calculated grazing coefficients attributable to calanoid copepods on co-occurring C. polykrikoides were up to 0.018 d−1 (i.e., up to 1.8% of the population of C. polykrikoides was removed by calanoids in a day). Furthermore, the calculated grazing coefficients attributable to calanoid copepods on co-occurring Prorocentrum spp. were up to 0.029 d−1 (i.e., up to 2.9% of the population of Prorocentrum spp. was removed by calanoid copepods in a day). Therefore, calanoid copepods may not control populations of C. polykrikoides or Prorocentrum spp. Furthermore, this maximum grazing coefficient is much lower than that by heterotrophic dinoflagellates and ciliates (Lim et al. 2017). In Masan Bay in 2004–2005, the grazing impact by the dominant calanoid copepod Acartia spp. on populations of Prorocentrum minimum was also much lower than that by the heterotrophic dinoflagellates and ciliates (Kim et al. 2013a, Yoo et al. 2013a). The abundance of copepod grazers is usually much lower than that of heterotrophic protist grazers although the ingestion rates of the former are greater than those of the latter. Thus, lower copepod abundance may be partially responsible for a lower grazing impact on populations of red tide dinoflagellates.
The calculated grazing coefficients attributable to total calanoid copepods on the co-occurring heterotrophic dinoflagellates Gyrodinium spp. were up to 0.047 d−1 (i.e., up to 4.6% of the population of Gyrodinium spp. were removed by total calanoids in a day). Furthermore, the calculated grazing coefficients attributable to total calanoid copepods on co-occurring Polykrikos spp. were up to 0.008 d−1 (i.e., up to 0.8% of the population of Polykrikos spp. were removed by total calanoids in a day). Therefore, calanoid copepods may not control populations of Gyrodinium spp. and Polykrikos spp.
During this study, the heterotrophic dinoflagellates Gyrodinium spp. were abundant during the red tides dominated by P. donghaiense (Jeong et al. 2017, Lim et al. 2017). Furthermore, the calculated grazing coefficients attributable to Gyrodinium dominans/G. moestrupii on co-occurring P. donghaiense were up to 0.58 d−1 (i.e., up to 44% of the population P. donghaiense were consumed in 1 d). Therefore, populations of P. donghaiense might be affected by the grazing of Gyrodinium spp., but the grazing impact could be lowered by the predation of calanoid copepods. Moreover, C. polykrikoides populations were affected by grazing of Polykrikos spp. (Lim et al. 2017). However, the grazing impact may not be lowered by predation of calanoid copepods.
Red tides have occurred in coastal waters of many countries (Holmes et al. 1967, Hallegraeff 1993, Anderson 1997, Sordo et al. 2001, Kang et al. 2013, Jeong et al. 2017). The results of this study suggest that red tides could affect the abundance of metazooplankton and in turn the grazing impact by metazooplankton could sometimes affect the abundance of red tide organisms in the South Sea of Korea. Thus, to understand interactions among red-tide organisms, heterotrophic protists, and metazooplankton, and the roles of heterotrophic protists and metazooplankton in the dynamics of red-tide organisms, the temporal and spatial variations in their distributions should be simultaneously investigated. Additionally, the grazing impact by heterotrophic protists and metazooplankton on populations of red tide organisms and in turn the predation impact on populations of heterotrophic protists should be quantified.
ACKNOWLEDGEMENTS
We thank Sang Pil Han, Seong Uk Kim, Young Seok Rho, Cheol Hee Kang for technical support. This research was supported by the National Research Foundation (NRF) funded by the Ministry of Science and ICT (NRF-2015M1A5A1041806; NRF-2017R1E1A1A01074419) and Pilot project for predicting the outbreak of Cochlodinium polykrikoides red tides funded by MSI (NRF-2014M4A1H5009428), the Useful Dinoflagellate Program of Korea Institute of Marine Science and Technology Promotion (KIMST) funded by the Ministry of Oceans and Fisheries (MOF) and Management of marine organisms causing ecological disturbance and harmful effect Program of KIMST, and Research Institute of Oceanography, SNU award to HJJ.